Iridescence as a result of interference
Although the iridescence of these natural wonders are a result of "structural colors," rather than pigment molecules, iridescence takes other forms as well. For example, light waves can interfere with one another, causing the iridescent colors you see in a layer of oil on a wet pavement or in the surface of a soap bubble.
Other examples of iridescence caused by interference include ice and crumpled cellophane, which will produce iridescent colors in polarized white light. Because they are optically anisotropic (they have different optical properties for light from different directions), they undergo double refraction. In contrast, the iridescent colors in opals, CDs, and DVDs that change with viewing angle is attributed to diffraction (the diffracted rays interfere to produce the colors).
What is interference?
Two light waves of the same wavelength can interact to reinforce each other if they are in phase, or cancel each other out if they are out of phase. Some cases of interference occur in combination with diffraction (see opal), while others involve interference in the absence of diffraction.
![]()
Constructive vs. destructive interference: light waves 1 and 2
produce constructive reinforcement if they are in phase (A). Crests
align with crests and troughs align with troughs, reinforcing one
another. Destructive cancellation is observed when they are out of phase
(B). The crests of wave 1 align with the troughs of wave 2, canceling
one another.
|
![]()
Interference of light beams reflected from the front and back
surfaces of a thin parallel film. This effect can be seen in soap
bubbles. As either the angle, the thickness of the film, or the
wavelength changes, the wavelength and the color of light produced by
constructive interference changes.
|
The ability of lasers to produce monochromatic light has opened up the study of interference, and led to the wide use of a variety of interference-based devices. Interferometers, such as the Twyman-Green interferometer and the Fabry-Perot etalon (using multiple reflective surfaces), are used for precise comparison of waves in applications ranging from oceanography to measurements at the microscopic scale.
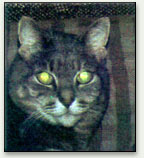
Green metallic-like reflection of a photographic flash from the eyes of a cat, caused by multiple thin-film interference.
Iridescence in the animal kingdom
Structural colors occur naturally in a rich variety of systems, usually involving multiple-layered structures that provide thin-film interference. The layers may be composed of keratin, chitin, calcium carbonate, mucus, and so on. Frequently, a dark layer of melanin forms a backing to intensify the observed color by absorbing the non-reflected light.The eyes of many nocturnal animals contain multilayer structures that improve night vision and produce iridescent metallic-like reflections.
Several species of stomatopod crustaceans, a family of tropical marine predators, have colored display surfaces that change dramatically when viewed with a linear polarizing filter. If the angle of polarization is changed, the uropods and antennal scales change from red-orange to faint purple. Researchers are studying the cause of this color (perhaps interference, perhaps a pigment), and whether the animals use these signals to communicate.
No comments:
Post a Comment